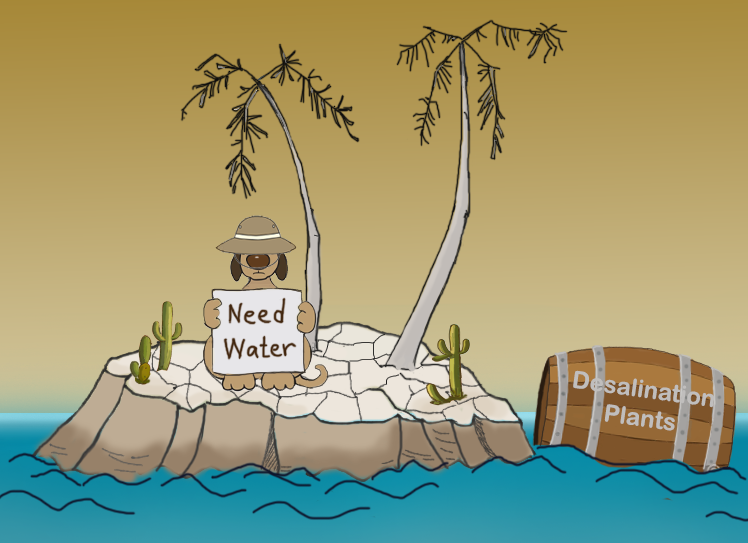
Water is an essential resource that everyone depends on for a variety of purposes on a daily basis. Unfortunately, many parts of the world are experiencing water crises; mainly, our freshwater supplies are dwindling. As we head into a future of uncertainty and increased climate variability, the question of how to maintain a stable freshwater supply has been increasingly posed and debated. Many have suggested desalination, the process of removing dissolved mineral salts from water, as a potential solution to this problem. While reservoirs, aquifers, or other sources of water may not be sufficient to provide for our growing populations, we have copious amounts of salt water in our vast oceans. In a way, “it represents a promise of near unlimited water supply and is an attractive potential solution to the age-old conundrum of seawater abundance and practical inaccessibility for potable use” (Kumar et al., 2017). What if there was a way we could use that saltwater supply and convert it into usable freshwater? Much research has been conducted on its effectiveness and impact; many countries employ desalination today. However, there has been much debate over whether or not it is the optimal solution to water scarcity, when taking into consideration its environmental consequences, economic implications, and realistic feasibility.
What is water scarcity?
Water scarcity is looked at as a “relative concept” (United Nations, 2021). As supply and demand change, so does its physical accessibility. Since water is a finite source and its demand increases, the scarcity is intensifying. This intensification is met with failure from countries and their lack of strong infrastructure and resources to meet the demand (United Nations, 2021). The important distinction between water scarcity and simply a scarcity in a physical resource is that water scarcity is “the availability of an acceptable quantity and quality of water for health, livelihoods, ecosystems and production, coupled with an acceptable level of water-related risks to people, environments and economies” (Grey & Sadoff, 2007). For example, water scarcity considers the extent to which water stress would impact a community that has no infrastructure, thus lacks a well integrated water resource management system. It considers the political interstate conflicts that may prevent certain areas from receiving water from their main sources.
In recent years, the combination of multiple factors has contributed to the acceleration of water scarcity. The distinguished technical staff member at Sandia National Laboratories Thomas E. Hinkebein would point to three: drought, climate change, and population growth (Hinkebein, 2004). With a larger population, more water is needed to sustain the population. That is a given. In areas like sub-Saharan Africa, the “water use is expected to double over the next twenty years” because of increasing demand for water-intensive crops like sugarcane and cotton (Penney & Muyskens, 2023). At the same time, changes in the climate are affecting our water supply. Our traditional precipitation and weather patterns are shifting, not only drying out certain regions and reducing water supplies (Penney & Muyskens, 2023) but also increasing its variability, making it difficult for agriculture. Examine the large states in America: Arizona, Nevada, Texas, New Mexico, and California. Arizona, Nevada have “40 and 60 percent growth rates per decade” respectively, and Texas, New Mexico, and California are in “dire need of water” (Hinkebein, 2004). Shockingly, even the water level of the largest freshwater body in the world, Lake Superior, has dropped 18 inches in the past decade (Hinkebein, 2004). Analyzing water scarcity and its trajectories reveals that “while water consumption increased fourfold within the study period, the population under water scarcity increased from 0.24 billion (14% of global population) in the 1900s to 3.8 billion (58%) in the 2000s” (Kummu et al., 2016). More disturbingly, almost all of the sub-national trajectories we have analyzed show “an increasing trend in water scarcity” (Kummu et al., 2016).
And unfortunately, this problem is affecting people all across the world. In fact, “two thirds of the world’s population experience severe water scarcity for at least one month each year. That’s roughly “four billion people” (UNICEF, 2020). And with climate change exacerbating this, fifty percent of the world’s population “could be living in areas facing water scarcity by as early as 2025” while around “700 million people could be displaced by intense water scarcity by 2030” (UNICEF, 2020).
At this rate, the question becomes: How can we possibly ensure that we can build a sustainable society that has the capacity to provide water to everyone?
Desalination: a possible solution?
It is simply the “removal of salt and contaminants from water” (Kumar et al., 2017). Essentially, it is the conversion of saline water into freshwater, which means that it needs a salt in water concentration of less than 1,000 ppm (Water Science School , 2019). This can be achieved through methods as simple as boiling saline water, capturing the steam, and distilling the gas back into liquid. However, in order to desalinate on a greater scale to provide for large populations, the process is more complicated, energy intensive, and expensive (Water Science School , 2019). Claude “Bud” Lewis Carlsbad Desalination Plant is North America’s largest desalination plant near San Diego, California, providing freshwater for around 3.1 million people in the region (Water Science School, 2019). The plant uses a desalination technique called reverse osmosis, which is essentially forcing saline water through a filter (or semi-permeable membrane). The larger salt molecules are filtered out while the smaller water molecules pass through the membrane holes.
In June of 2015, 18,426 desalination plants were operating around the world, producing around 86.8 million cubic meters per day which is enough water to provide for around 300 million people. In two years, there has been a 10.71% increase as in 2013, desalination plants produced 78.4 million cubic meters. Countries of the Middle East such as Saudi Arabia, Kuwait, the United Arab Emirates, Qatar and Bahrain as important locations for desalination plants, using about “70% of worldwide capacity” (International Desalination Association, n.d.). And this makes sense because these are the regions with the highest levels of water stress. The United States, especially California and Florida, also heavily uses desalinated water. In fact, 11% of California’s total water use in 2015 was from saline surface water and saline groundwater (International Desalination Association, n.d.).
Interestingly, desalination is not a novel process. One could even look at desalination from a less anthropogenic view: even evaporation, condensation, and other natural water processes are essentially desalination (Angelakis et al., 2021). It’s all around us at all times.
Although modern-day approaches to desalination involve more advanced technologies, ancient societies have long implemented it using a broad range of methods. In fact, its “long history in both mythology and practice” (Kumar et al., 2017) is exemplified by its reference to the Bible (Exodus 15:22-26). Aristotle also thought profoundly about the removal of salt from water through filters as early as 350 B.C.E (Aristotle, 350 B.C.E). When examining the Mediterranean Sea, for thousands of years, the Minoan sailors implemented desalination by boiling and evaporating the water to separate the salt concentrations.
It wasn’t until the Industrial Revolution when technology paved the way for the “modern era of desalination” as “implementation of this idea on a large-scale… would also be economically enticing” (Babak Zolghadr‐Asli et al.). Curaçao, Netherlands Antilles was the first place to construct a land-based distillation plant in 1928, supplying 60 cubic meters of potable water per day. Countries like Saudi Arabia, with challenges to accessing water, followed this by creating multi-effect distillation plants, realizing the potential in this innovation. The Second World War was a key driving force in the implementation of desalination; afterwards, countries all over the world resorted to this technology as means of solving their water scarcity issues, especially in remote arid regions or areas with increasing municipal demand. It soon became a prioritized “common practice” rather than an intriguing concept or idea (Babak Zolghadr‐Asli et al.).
So, what’s holding us back from desalination?
Clearly desalination has played and continues to play a prevalent role in our lives already. So why is it part of the discussion now? Why don’t we just continue to implement it? Well, there are many reasons, actually. Sure—in theory, it would work. Take an infinite supply of salty water, and convert it to usable freshwater. Now, it appears we have solved the water crisis. Unfortunately, the demand for water has reached unprecedented levels and thus the costs that come with this process are amplified as well. Desalination has huge environmental impacts that are important to take into consideration in this conversation. Desalination plants are extremely wasteful and produce brine, a waste product. In fact, around 141.5 million cubic meters of brine are produced for every 95 million cubic meters of freshwater in these plants; the brine contains chlorine, copper, and other toxins that are then dumped into natural bodies of water. This is extremely harmful to the environment because water with lowered oxygen concentrations or overly salty water poses immense threats to marine life (Williams.) With a “salinity at least 1.6 — 2.1 times higher than seawater,” brine poses significant threats to the ecosystems around them, such as cell dehydration. As a result, fishes, plankton, algae, seagrass, and other marine species experience “lethal osmotic shock” (Abushaban, 2019; Levitt, 2015; Panagopoulos & Haralambous, 2020).
Some might argue that some marine organisms, like the euryhaline teleost fish, can modify their cell and tissue functions to tolerate a wide range of salinities and salinity changes in the environment (Inokuchi et al., 2021), rather than focusing on select species that have the incredible ability to adapt to dramatically changing environments, it’s important to look at this problem holistically. Overall, “the abundance of fauna and flora will be affected, and in some occasions, changes in the ecosystem may attract other unusual species in the region under normal conditions.” In addition, the magnitude of the impact of desalination varies based on the characteristics of both the brine produced and the environment it is discharged into. Interestingly, the most affected environments are “closed, semi-closed and shallow places, with an abundance of marine life,” such as the Red Sea and Mediterranean Sea (Panagopoulos & Haralambous, 2020).
Furthermore, that was just one environmental impact of desalination. Critics would point to the extreme energy consumption desalination partakes in. For example, for every million gallons of fresh water produced, the plants use 15000 kilowatt-hours of power. Most plants use diesel to fuel their power pumps and in the ongoing discussion of fossil fuels and climate change, this is not exactly the most appealing (Williams).
The reverse osmosis process is unfortunately also very expensive. That is why the freshwater produced by Claude “Bud” Lewis Carlsbad Desalination Plant is twice as expensive as other water sources (Water Science School , 2019). This poses economic barriers for countries who don’t have the infrastructure nor money to build these plants. In fact, the “cost of treating seawater is about $2,000 to $3,000 an acre foot” which is double or triple the cost of water conservation efforts (Jacobo, 2022). The world’s wealthiest countries carry out 90% of seawater desalination. Weaker economies are faced with these economic barriers due to the high investment costs to build and run expensive plants to produce high costing drinkable water (Desalination and Its Challenges, 2023).
There are also health concerns about the safety of desalination water. Research shows that drinking desalinated water “can lead to electrolyte abnormalities marked by hyponatremia, hypokalemia, hypomagnesemia and hypocalcemia which are among the most common and recognizable features in cancer patients” (Nriagu et al., 2016). Many scientists call for further research to be conducted to examine the impact of electrolyte abnormalities on the development of cancer (Nriagu et al., 2016).
Overall, there has been a lot of debate on whether or not desalination is the solution to water scarcity. Many oppose it for its detrimental impact on the environment. Many others are against the economic inequities associated with the installation of these plants. So, what do opponents of desalination propose?
Alternatives to Desalination
Considering the detrimental ecological, economic, and health impacts of desalination plants, there are many proponents to alternative approaches such as water conservation. Water conservation can be achieved in various ways. Stormwater capture and reuse and recycled water expansion are just some examples of them. In the agricultural field, micro-sprinklers and drip irrigation can replace flood irrigation (Penney & Muyskens, 2023). Drip irrigation is much more efficient and saves “up to one-fourth of the water used is saved, as compared to flood irrigation” (USGS, n.d.). In addition, these alternatives “provide pollution abatement, habitat restoration, and flood control benefits, which are commonly overlooked during cost/benefit assessments” (Heal The Bay, n.d.).
There could also be less pressure put on the people to conserve water, and more pressure to enact systemic change. For example, “cities can develop infrastructure to capture and reuse stormwater runoff, repair leaks in municipal water systems and encourage water efficiency” (Penney & Muyskens, 2023). And this has been done before. The Las Vegas Valley Water District in Nevada took measures to create a grass replacement program and began imposing fines on water waste. The results were tremendous: their total water use was reduced by “26 billion gallons per year from 2002 to 2021, even as the area’s population grew by 750,000 residents” (Penney & Muyskens, 2023). Legislations that address root issues could be enacted. The EPA has passed water legislations in the past, including Clean Water Act (CWA) and the Federal Water Pollution Control Act of 1948. Policy makers should take into consideration the driving factors behind water scarcity in a certain region and advocate for policy changes in that sector.
Optimal Approach to Tackling Water Scarcity
In certain areas, desalination is a necessity to provide a stable water supply, especially in water scarce regions. Yes, there are drawbacks to desalination. That’s why as important as it is to acknowledge the drawbacks, it’s just as important not to neglect the benefits it can bring to a region. Moreover, there are going to be drawbacks to nearly every approach or change we make to keep humanity alive. And the issue with water scarcity and climate change is that we don’t have any time, especially for those who are marginalized or those who are disproportionately affected. Take a look at Gaza. As the last seawater desalination plant shut down, it raised fears from the people about water scarcity and clean water running out as Israeli airstrikes attacked the Palestinian territory. Without access to clean water, people are at higher risk of water diseases such as cholera or could potentially dehydrate to death. These people don’t have a choice, and at some point, it is necessary to weigh the benefits and drawbacks and realize that there are already people immediately impacted by water scarcity. Installing and investing in desalination plants in these regions is not a proposal; it is a necessity. (McKernan, 2023) In Gaza, less than 3% of the water in their aquifer is actually potable water. And the consequences of not having sufficient water are dire, as discussed earlier. Health effects include dehydration, which has serious health complications. For example, there is an increased risk of heat injury, urinary and kidney problems, seizures, and in extreme cases, low blood volume shock (hypovolemic shock) (Mayo Clinic, 2021). The kidneys could shut down, eventually leading to a coma or death, or even permanent organ or brain damage (McKernan, 2023). As it poses life threatening consequences, water scarcity is of utmost concern in relation to public health and safety.
Then again, it’s critical not to neglect the consequences of installing desalination plants. There are severe environmental consequences that come with these plants as mentioned earlier like brine, toxic chemical pollution, and excessive energy use.
Just as the magnitude of the impact of desalination differs by region and by species, it’s important to approach these problems with specific solutions case by case. Although desalination has serious drawbacks, some regions do not have the luxury of time to try alternative solutions. Thus, leaders or governments of regions should follow through a pyramid or step-by-step evaluation of the situation of the specific population that weighs the costs and benefits and short and long term impacts. It would incorporate a similar concept to an Integrated Pest Management (IPM) triangle which prioritizes cultural, physical, biological, and chemical practices in order. Chemical practices are the last resort because they have the most severe ecological consequences. In addition to this order of practices, the evaluation should take into consideration how urgently the water is needed and the extent to which it is needed. We should redirect our focus to reducing the impact so that water-scarce regions can get the water they need. For example, we can use mitigation methods for brine, such as “Near-field and far-field modeling approaches or Environmental monitoring plans (EMPs)” (Panagopoulos & Haralambous, 2020).
What’s more, we can even work on improving desalination processes to reduce their environmental impacts and reduce the severity of economic barriers they impose. Technological innovation is advancing rapidly, and this could benefit desalination. Already, MIT and China’s engineers are working to create a solar powered desalination device the size of a small suitcase and inspired by the ocean’s functions (Chu, 2023). This also highlights our need to invest and divert our resources to the right activities. More research needs to be conducted on efficient renewable ways to power processes that alleviate climate stress in general; desalination could be one of them.
References
Angelakis AN, Valipour M, Choo K-H, Ahmed AT, Baba A, Kumar R, Toor GS, Wang Z.
Desalination: From Ancient to Present and Future. Water. 2021; 13(16):2222.
https://doi.org/10.3390/w13162222
Aristotle. “Meteorology.” Internet Classics Archive. (June 18, 2010)http://classics.mit.edu/Aristotle/meteorology.2.ii.html
Babak Zolghadr‐Asli, et al. “A Closer Look at the History of the Desalination Industry: The Evolution of the Practice of Desalination through the Course of Time.” Water Science & Technology: Water Supply, vol. 23, no. 6, UWA Publishing, June 2023, pp. 2517–26, https://doi.org/10.2166/ws.2023.135.
Balsom, P. (2020, September 28). Water Usage In The Agricultural Industry. High Tide. https://htt.io/water-usage-in-the-agricultural-industry/#:~:text=How%20Much%20Water%20Does%20The
California Department of Water Resources. “Drought.” Ca.gov, 2015, water.ca.gov/Water-Basics/Drought.
California Invests in Desalination Projects and Research to Help Diversify Local Water Supplies for Communities. (2023, April 19). Water.ca.gov. https://water.ca.gov/News/News-Releases/2023/April-23/California-Invests-in-Water-Supply-and-Research-to-Diversify-Local-Water-Supply
Chu, J. (2023, September 27). Desalination system could produce freshwater that is cheaper than tap water. MIT News | Massachusetts Institute of Technology. https://news.mit.edu/2023/desalination-system-could-produce-freshwater-cheaper-0927
David Grey, Claudia W. Sadoff; Sink or Swim? Water security for growth and development. Water Policy 1 December 2007; 9 (6): 545–571. doi: https://doi.org/10.2166/wp.2007.021
https://www.ncbi.nlm.nih.gov/pmc/articles/PMC9029386
Desalination and its challenges. (2023, January 4). We Are Water. https://www.wearewater.org/en/desalination-and-its-challenges_356201
Department of General Services (DGS). Https://Www.dgs.ca.gov/-/Media/Divisions/OS/ADA-Checked/Bestpracticeswater-2021.Pdf.
Heal The Bay. (n.d.). Ocean-Water Desalination: A Solution or a Problem? https://healthebay.org/sites/default/files/Desalination%20FAQ%20Sheet_final.pdf
Hinkebein T. Desalination: Limitations and Challenges. In: National Research Council (US)
Chemical Sciences Roundtable; Norling P, Wood-Black F, Masciangioli TM, editors. Water and Sustainable Development: Opportunities for the Chemical Sciences: A Workshop Report to the Chemical Sciences Roundtable. Washington (DC): National Academies Press (US); 2004. 4.
Inokuchi M, Yamaguchi Y, Moorman BP and Seale AP (2021) Age-Dependent Decline in Salinity Tolerance in a Euryhaline Fish. Front. Aging 2:675395. doi: 10.3389/fragi.2021.675395
International Desalination Association. (n.d.). Desalination at a glance. https://idadesal.org/wp-content/uploads/2021/06/desalination-at-a-glance.pdf
Jacobo, J. (2022, May 13). California panel unanimously rejects proposal for plant to turn ocean water into drinking water. ABC News. https://abcnews.go.com/US/california-panel-unanimously-rejects-proposal-plant-turn-ocean/story?id=84671584
Mayo Clinic. (2021). Dehydration – symptoms and causes. Mayo Clinic. https://www.mayoclinic.org/diseases-conditions/dehydration/symptoms-causes/syc-20354086
Nriagu J, Darroudi F, Shomar B. Health effects of desalinated water: Role of electrolyte disturbance in cancer development. Environ Res. 2016 Oct;150:191-204. doi: 10.1016/j.envres.2016.05.038. Epub 2016 Jun 10. PMID: 27295409.
Panagopoulos, A., & Haralambous, K.-J. (2020). Environmental impacts of desalination and brine treatment – Challenges and mitigation measures. Marine Pollution Bulletin, 161, 111773. https://doi.org/10.1016/j.marpolbul.2020.111773
Penney, V., & Muyskens, J. (2023, August 16). Here’s where water is running out in the world — and why. Washington Post. https://www.washingtonpost.com/climate-environment/interactive/2023/water-scarcity-map-solutions/
Popkin BM, D’Anci KE, Rosenberg IH. Water, hydration, and health. Nutr Rev. 2010
Aug;68(8):439-58. doi: 10.1111/j.1753-4887.2010.00304.x. PMID: 20646222; PMCID: PMC2908954.
United Nations. (2021). Water Scarcity. UN-Water; United Nations. https://www.unwater.org/water-facts/water-scarcity
USGS. (n.d.). Irrigation: Irrigation techniques, USGS Water-Science School. Water.usgs.gov.
Retrieved March 4, 2024, from https://water.usgs.gov/edu/irmethods.html#:~:text=Drip%20Irrigation%3A&text=Water%20is%20sent%20through%20plastic
“Water Desalination: History, Advances, and Challenges – Manish Kumar, Tyler Culp, and
Yuexiao Shen.” National Academy of Engineering. 2017. Frontiers of Engineering: Reports on Leading-Edge Engineering from the 2016 Symposium. Washington, DC: The National Academies Press. doi: 10.17226/23659.
Water Science School . (2019, September 11). Desalination | U.S. Geological Survey. Www.usgs.gov. https://www.usgs.gov/special-topics/water-science-school/science/desalination
Williams, Kori. “The Desalination Process Gives Us Freshwater – at a Huge Environmental
Cost.” World Economic Forum, 16 Dec. 2022, www.weforum.org/agenda/2022/12/desalination-process-freshwater-negative-environmental-cost/#:~:text=Desalination%20removes%20salt%20from%20saltwater.
The views and opinions expressed are those of the authors and do not necessarily reflect nor represent the Earth Chronicles and its editorial board.